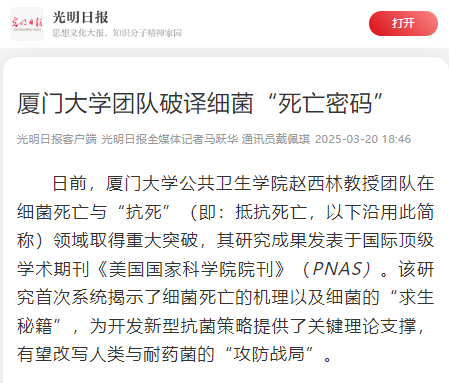
This article is republished from Guangming Daily:
Recently, Professor Xilin Zhao’s team from the School of Public Health at Xiamen University has made a groundbreaking breakthrough in the field of bacterial death and "anti-death" (resistance to death, hereafter referred to as "anti-death"). Their research findings, published in the top international academic journal Proceedings of the National Academy of Sciences (PNAS) USA, systematically revealed, for the first time, the mechanisms of bacterial death and their "survival playbook," providing critical theoretical support for developing novel antimicrobial strategies to fight drug-resistance bacterial infections. This discovery is poised to reshape the battle between humans and drug-resistant bacteria.
Life and death are two equally fundamental processes for all living organisms, yet human understanding of these phenomena remains highly asymmetrical. Decades of research have yielded profound insights into the principles of "life," but the mechanisms of death remain poorly understood. This knowledge gap arises because death occurs rapidly, often eluding observation, and once the death process is completed, organisms enter a passively inactive "dark box" where conventional methods for studying active biological processes become ineffective. To overcome these technical barriers, Professor Zhao’s team proposed a revolutionary approach: instead of directly studying death, they focused on how life resists death under extreme conditions, then reverse-engineered the molecular pathways of death itself through dissecting "anti-death" activities. The team developed a high-throughput screening model for "anti-death" mutants and identified bacterial variants capable of surviving lethal phenol disinfectant exposure. This breakthrough unlocked the molecular "dark box" of bacterial death and anti-death mechanisms.
The study found that bacteria with defects in the ptsI gene (a key component of the carbohydrate transport phosphotransferase system, PTS) can activate a "metabolic emergency brake" under diverse death-inducing stresses. By switching from high-speed to energy-saving metabolic modes, these bacteria shut down efficient sugar metabolism pathways, reducing the accumulation of reactive oxygen species (ROS), a lethal byproduct of rapid metabolism, thereby suppressing cell death. Further molecular investigations revealed that PtsI phosphorylation activates the synthesis of cyclic adenosine monophosphate (cAMP), a "death signal molecule." Elevated cAMP triggers hypermetabolism, leading to excessive ROS accumulation and bacterial death. This study is the first to demonstrate the existence of a universal PTS-cAMP-Crp (cAMP-binding protein) regulatory pathway that governs bacterial death and is applicable to nearly all bactericidal agents that rely on ROS for killing bacteria.
Building on the discovery of the cAMP-mediated death pathway, the team further identified that the novel pheS F158C mutation disrupts protein synthesis accuracy and speed, triggering a "global (general) stress response" in bacteria. This response generates high levels of guanosine tetraphosphate (ppGpp), an "anti-death signal molecule." Elevated ppGpp reprograms bacterial transcription, promoting the production of antioxidant enzymes, repairing damaged DNA, suspending non-essential metabolism to conserve energy, and reducing ROS accumulation, which collectively forms a multi-layered defense network to suppress cell death.
This research not only elucidates the existence of universal pathways governing bacterial death and anti-death, but it also identifies key signaling molecules in these processes. It offers innovative strategies for combating bacterial threats. For example, stimulating the cAMP death pathway could enhance pathogen eradication, improve the efficacy of anti-infection therapies, and reduce development of antibiotic resistance. Conversely, activating the ppGpp anti-death pathway could protect beneficial probiotics and engineered industrial strains used in microbial factory from harsh environmental conditions during late-stage cultivation.
The study was led by Professor Xilin Zhao of Xiamen University, with doctoral candidate Miaomiao Chen and master’s student Runbo Cui as co-first authors. Collaborators include Professor Jianjun Niu from Xiamen University Affiliated Zhongshan Hospital and Professor Yuzhi Hong from Soochow University. The research was supported by the National Natural Science Foundation of China and the China Postdoctoral Science Foundation.
Report Link:
https://app2.gmdaily.cn/as/opened/n/d86167b090514496ad30074e1cd5de8e(From the Guangming Daily)
https://fjrb.fjdaily.com/pc/con/202502/22/content_432063.html(From the Fujian Daily)